重力波プロジェクト推進室長 ラファエレ・フラミニオ博士 インタビュー [2/4]
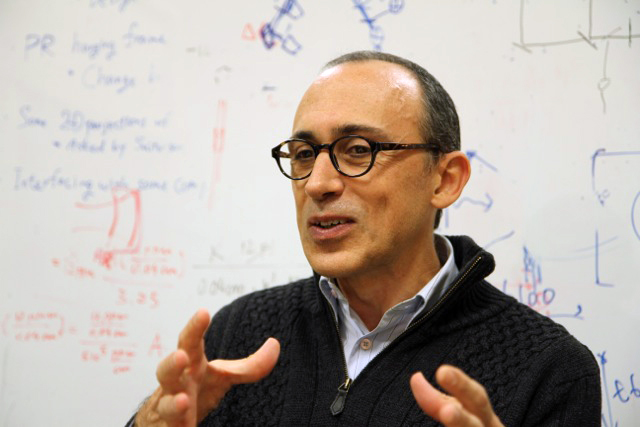
――重力波は光ではありません。重力波はどのようにして検出されるのでしょう?
Gravitational waves are not light. How do you detect gravitational waves?
Flaminio:重力波というのはつまり、基本的には時空の形の変化です。言い換えると、重力波は時空に浮かんでいる物体間の距離を相対的に変化させます。ですから、重力波を捉えるには、物体間の距離を測れば良いのです。
So a gravitational wave is basically a change in the geometry of space-time. In other words a gravitational wave produces a change in the relative distances between free-floating objects. So the way to detect this is to be able to measure these distances.
この距離の変化を、二つの質量の間に潮汐力に似た相対的な力が働いている、と理解することもできます。このため、重力波を検出する最初の実験装置として、長さ数メートルの金属棒でキロヘルツ(kHz)前後の機械共振周波数を持つ共振器が組み立てられました。重力波が共振器を通過すると、エネルギーの一部を共振器に与えるので、共振器の固有振動が励起される、という考えに基づいてつくられています。音叉や鐘をたたくと、その音が長く響いて徐々に消えていくように、重力波によって共振器固有の振動が励起され、減衰していくのを見ることで重力波をとらえることができます。共振器型の重力波検出器は、長い間開発が続けられていますが、まだ実際に重力波を検出するのに十分な感度には達していません。
This change in distance means also that there is a relative force between these 2 masses, which is a kind of tidal force. So the first attempt to detect gravitational waves consisted of building resonant bars a few meters long, which had some internal resonances around kilohertz or so. And the idea was that as the gravitational wave passes by the bar, the gravitational wave leaves some energy in the bar and excites the resonance. So you should see the decay of the excited motion in the internal vibration of this bar, like after striking a tuning fork or a bell. People had been developing these resonant bars for many years, but the sensitivity was not good enough to actually detect a signal.
60年代からは、レーザー干渉計型とよばれる検出器の検討がはじまりました。これはレーザーを使って物体間の距離を測る装置です。
And so from the 60s, people started thinking of another system which consisted of using a laser interferometer; which means measuring the distance between objects by means of a laser.
実際には、重力波による距離の変化は方向によって変わります。重力波の進む方向に垂直な面上のある方向で距離が減少すると、それに垂直な方向では距離が増加します。マイケルソン型のレーザー干渉計を用いることで、こうした距離の変化を測ることができます。
Actually these waves are such that the change in distance depends on the orientation of the wave. The distance decreases in one direction, and increases in the direction perpendicular to that. And so by using a device called a Michelson laser interferometer, one can measure exactly the particular shape of the changes in distance.
レーザー干渉計型の検出器で達成できる精度について初めて現実的に評価したのはマサチューセッツ工科大学のライナー・ワイス *1でした。70年代初頭のことですが、そのすぐ後に最初の干渉計が作られました。およそ1.5mの基線長のごく基本的な干渉計で、信号は電気的に増幅され、いわゆるデータ解析は実際に干渉計からの信号をヘッドホンで聞いて何が聞こえるかと耳をすませることでした。ロバート・L・フォワードが1970年代にこの実験を行いました。
The first real study to evaluate what is the precision which one can achieve with such an instrument came from Rainer Weiss at MIT. This was at the beginning of the 70s, and shortly after that the first interferometer was built. This was a very basic interferometer, around 1.5 m long, where the signal was amplified electronically and the so called data analysis actually consisted of listening to the signal of this interferometer with headphones to see if we could hear something. This was done by Robert L. Forward, in the 1970s.
その後、研究者たちは干渉計の感度を上げるために、もっと長い干渉計をつくりはじめました。80年代には、アメリカとヨーロッパに30 mから 40mくらいの干渉計が作られました。そして90年代には、数100mの干渉計が作られました。ここ国立天文台にも基線長300mの干渉計が建設されました。そしてTAMA300 *2は、世界ではじめて共振型の検出器を上回る感度を実現したレーザー干渉計なのです。TAMA300の成功によって共振型の検出器は主流ではなくなり、本当の意味で、レーザー干渉計によって重力波を捉えようとする試みが始まったのです。
After that, people started trying to increase the length of the interferometers. In the 80s, we had interferometers around 30 or 40 meters in the United States and in Europe. And then in the 90s, people started scaling to hundreds of meters. An interferometer 300 meters long was built here at NAOJ. And actually this interferometer, TAMA300, was the first to have a sensitivity better than these resonant bars. So that was the end of the resonant bars and really the beginning of attempting to detect gravitational waves with laser interferometers.
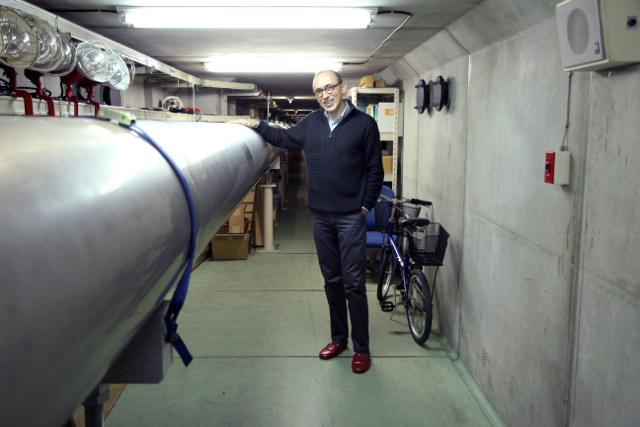
世界初の高感度レーザー干渉計型重力波検出器、TAMA300のトンネルにて
TAMAの建設とほとんど同時期に、アメリカではLIGO *3が承認されました。ヨーロッパでは、ドイツとイギリスによってGEO600 *4が、そしてフランスとイタリアの研究機関によってVIRGO *5が承認されました。基線長4kmのLIGOと3kmのVIRGOによってキロメートルクラスのマイケルソン型干渉計の建設が始まったのです。
At more or less the same time that TAMA was being built, the Americans approved the LIGO project. And in Europe, the GEO600 project was approved by the German and the British; and the VIRGO project was approved by the French and the Italian research funding institutes. So the construction of kilometer long Michelson interferometers started with LIGO and VIRGO which are 4 and 3 kilometers long, respectively.
LIGOとVIRGOの感度なら、一回くらいは重力波を検出できてもよさそうでした。必ず検出するとまではいえませんが、少なくとも検出してもおかしくはない感度です。ですから、これらの検出器によって重力波天文学がはじまったといってよいでしょう。
With LIGO and VIRGO the sensitivities were such that a detection was considered as possible. Maybe not very probable, but at least possible. So that was really the beginning of gravitational wave astronomy research.
その間に日本では、CLIOの開発が進められていました。CLIOは基線長こそ100メートルですが、干渉計型検出器の観測精度を制限する鏡の熱振動を低減するための低温鏡を備えていました。このCLIOとTAMAの経験に基づいて、日本の研究者はKAGRA *6(正式名称は「大型低温重力波望遠鏡(LCGT)」)の建設を求めていました。KAGRAは2010年に承認され、現在建設を進めていますので、じきにLIGOやVIRGOと共にデータを取得できるようになるでしょう。Advanced LIGO(LIGOを改良して感度を向上させた装置)、Advanced VIRGO、KAGRAのような高い感度の望遠鏡によって、今まさに世界ではじめて重力波が直接検出されようとしているのです。
In the meantime in Japan, there was the development of CLIO, which was an interferometer 100 m long, but had cryogenic mirrors to reduce the thermal vibrations of the mirrors which limit the precision. And based on that experience with CLIO and also the experience with TAMA, Japanese researchers proposed the KAGRA project (LCGT “Large Cryogenic Gravitational-wave Telescope” was the original name). KAGRA was approved in 2010; we are building it now and we hope to be able to take data soon, together with LIGO and VIRGO. Now we are really at the point where the sensitivities which we are going to have with Advanced LIGO, Advanced VIGRO, and KAGRA, should really lead to the first detection.
重力波望遠鏡の改良の努力と平行して、天文学者たちが中性子星やブラックホール、パルサーについて多くの新しい観測を進めてくれました。おかげで、私たちは宇宙に存在する重力波源候補天体についての知見を深めることができました。
In parallel with this process of improving the detectors, there have been a lot of new observations made by astronomers about neutron stars, about black holes, about pulsars that have allowed us to have a better understanding of what we can expect in terms of gravitational wave sources in the Universe.
――KAGRAの参加はこの国際観測網にどのようなメリットをもたらすのでしょうか?
What advantages does KAGRA add to this international collaboration?
Flaminio:LIGOとVIRGOは1990年代に建設が始まりました。これらの干渉計は数キロメートルの基線長をもつ重力波望遠鏡としては世界で初めてのものでした。このように長い干渉計を作ること自体が世界で初めてのことだったので、他の点ではいくらか保守的に作られています。
LIGO and VIRGO detectors were built starting from the 1990s. They were the first gravitational wave detectors with arms kilometers long. Since it was the first time that we were doing something like that, some of the choices were conservative, let’s say.
KAGRAはLIGOやVIRGOから15年以上経ってからようやく資金の目途が立ちました。そろそろ、もう少し野心的な挑戦を始めなければなりません。そこで、まず地下に建設することにしました。地中深くに入るほど、雑音となる地面の振動が小さくなるからです。2つ目の挑戦として、鏡を低温に冷やすことにしました。どれくらい冷やすかとういうと、-253℃ほどの極低温までです。もちろん、これはとても野心的な試みですが、これらの選択によって、より高い感度を達成することができます。
KAGRA was funded more than 15 years after LIGO and VIRGO. The time had come to do something a little bit more ambitious. And so it was decided to build it underground because the seismic vibrations are lower as you start going underground. And the second thing was to try to build an interferometer where the mirrors are cooled down to cryogenic temperatures. To something like -253 degrees below 0 Celsius. Of course this is rather ambitious, but it allows us to attain a better sensitivity.
ですからKAGRAは、はじめは、Advanced LIGOやAdvanced VIRGOに近い感度になるでしょうが、地下で、鏡を低温で機能させることができれば、将来的にはAdvanced LIGOやAdvanced VIRGOより良い感度を到達することができるかもしれません。
So KAGRA, in the first stage, will have a sensitivity similar to Advanced LIGO and Advanced VIRGO, but then with this underground site and this possibility to operate the mirrors at cryogenic temperatures, it has the potential to go beyond the sensitivity of Advanced LIGO and Advanced VIRGO.
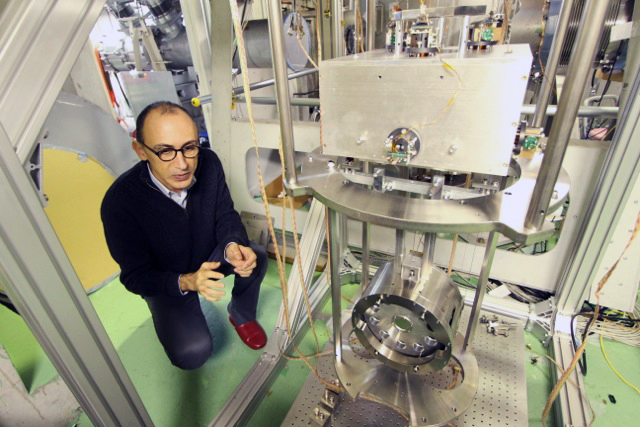
TAMA300で培われた防振の工夫がKAGRAに繋がっていった。
実は、ヨーロッパでも同じようなことが考えられています。ヨーロッパではすでに重力波の初検出後を見据えてアインシュタイン望遠鏡(Einstein Telescope; ET)とよばれる新しいプロジェクトの議論がはじまっています。私もETに関わっています。私たちが提案しているこの計画では、基線長30キロメートルの干渉計を三角形状に作ります。三角形のそれぞれの頂点に鏡とレーザーを設置します。そしてこの三角形の二辺ずつを使って、お互いに他の2つの干渉計と重なる部分を持つ3つの干渉計を作ることができます。こうすることで、3つの干渉計で重力波を同時に検出することができるようになります。重力波の偏波は2種類あって、1台の検出器では偏波を決めることはできませんが、3つの干渉計を同時に使うことで偏波についても調べることができるようになります。アインシュタイン望遠鏡を、KAGRAのように地下に作って、低温で動かすことも考えられています。つまりKAGRAのあるこの日本では、LIGOやVIRGOの建設期には時期が早すぎて採用できなかった新しい方法を選ぶことができるのです。
Actually something similar will happen in Europe where people are already discussing about a new project, called the Einstein Telescope, for after the first detection of gravitational waves. I was involved in that actually. The plan that we proposed was to build a 30 km long interferometer in the shape of a triangle. With a triangle you have 3 locations were a mirror and laser are placed. And by using this triangle, basically you can have 3 interferometers, nested one into the other two. And this should allow us to make a coincidence detection between these 3 interferometers. And also to have all the information about the properties of the gravitational wave which can be polarized in different manners. But they are thinking about building it underground and having the interferometer operate at cryogenic temperatures, like KAGRA. So here in Japan with KAGRA, we are opening these new options that LIGO and VIRGO could not implement because it was too early at the time that the projects were put in place.
――重力波は一世紀前に予言されましたが、まだ直接検出できたという話は聞きません。重力波の研究者はなぜこれほど長い間興味を失わずにいることができるのでしょうか?
Gravitational waves were predicted 1 century ago, but we have not yet been able to detect them directly. How do gravitational wave researchers stay motivated?
Flaminio:良い質問ですね。そうですね、目標がとても重要だから、と言うことはできるでしょう。重力波信号の検出は、基礎物理と天文学の境界線上にあります。重力波を検出することで、わたしたちは宇宙について学ぶことができる、と同時に、とても基本的なこと-重力や時空の性質-について学ぶこともできます。また次のようなことも考えられます。装置開発に携わる技術者や研究者たちには、おそらく実験の進展それ自体がとても面白かったのではないでしょうか。装置に関わる人たちは、量子光学、低損失機械工学、大きな高反射率鏡、超高真空系、低温技術、そして低雑音電子器機などに取り組んでいます。
It’s difficult to say. On one side, the fact that the goal is very important because the detection of this signal is really at the boundary between fundamental physics and astronomy. You can learn about the Universe, but also at the same time learn about fundamental things like gravity and the properties of space-time. On the other hand, probably also the fact that the experimental development itself was very interesting to instrumentalists, physicists working on instruments. There are people working on quantum optics, on low loss mechanics, on large high-reflectivity mirrors, on high vacuum, on cryogenics, on low noise electronics and so on.
たとえば鏡の場合、わたしたちは直径20 cm以上、質量およそ40 kgのレーザー鏡を作っています。そして、完全に平らな鏡をつくるための技術を開発しています。私の言う「平ら」とは、表面の凹凸が1 nm以下ということです、これは、東京が同じくらい「平ら」であれば、髪の毛ほどの凹凸も許されないという精度です。ですから本当に完全な研磨技術が必要なのです。それから、この平らな鏡面に、照射した光の99.9999%を反射する完璧な被膜を重ねるのです。
For example in the case of the mirrors, we are building laser mirrors over 20 cm in diameter, which are about 40 kg in mass. And we have been developing technology to have perfectly flat mirrors. When I say flat, it means that the variation of the surface should be much smaller than 1 nm, which means like if Tokyo was flat to the precision of a hair. So you really need to have a perfect polishing method; and then to deposit on this surface a nearly perfect reflector which sends back 0.999999 of the light you shine on the mirror.
このように、重力波の検出には多くの挑戦的な課題があります。そしてこうした挑戦があるからこそ、いろいろな科学者が興味を持ち続けているのでしょう。
There were so many challenges like this, that simply having these challenges keeps the motivation of many different kinds of scientists.
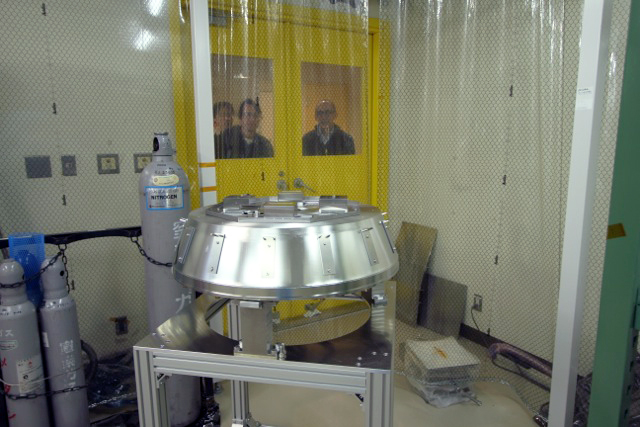
先端技術センターのクリーンルームで開発が進むKAGRAの装置。重力波の検出は機器開発にとっても意義深い挑戦だ。
時に、さまざまな人たちが一緒に働くことそれ自体が問題になります。私たちは往々にして、何が重要で、何が重要でないか、私たちはどうすべきか、といったことについて、違う見方をします。小さな実験室の中で、光学定盤上に組み立てた試験機で量子光学を研究していた人たちが、粒子加速器や巨大な粒子検出器を作っていた人たちと一緒に働くこともまた容易なことではありませんでした。天文学者と一緒に働くときも、文化が違うので、問題が起こることがあるでしょう。
Sometimes, one of the issues was actually to make all these people work together. Because they have sometimes different perspectives about what is important, what is not important, how we should work. It was also a challenge to see people who have been working in a small laboratory on a table-top prototype quantum optics experiment starting to work together with people who have built a particle accelerator or big particle detectors. Or working together with an astronomer which is also another community.
*1. ライナー・ワイス (Rainer Weiss, 1932-):ドイツ生まれの物理学者。マサチューセッツ工科大学名誉教授。レーザー干渉計による重力波検出法の基礎を確立し、LIGOプロジェクトの実現にも大きく貢献した。
*2. TAMA300:1995年に国立天文台三鷹キャンパスに建設された基線長300mの干渉計型重力波望遠鏡。欧米のkmサイズの干渉計の建設に先駆けて1000時間連続観測を達成するなど、その後の大型重力波望遠鏡の高感度観測に向けた技術開発をリードした。
*3. LIGO:1999年に完成したレーザー干渉計型重力波観測所(Laser Interferometer Gravitational-Wave Observatory)。カリフォルニア工科大学とマサチューセッツ工科大学の共同運用。基線長が4キロメートルの2つの重力波検出器をワシントン州ハンフォードとルイジアナ州リビングストンに有する。
*4. GEO600:ドイツのハノーファーに位置する基線長600メートルのレーザー干渉計型検出器。2002年から運用を開始。マックスプランク研究所とライプニッツ・ハノーファー大学の共同運用。
*5. VIRGO:イタリアのカーシナに位置するヨーロッパ重力研究所内に設置された基線長3kmのレーザー干渉計型重力波検出装置。フランスとイタリアの機関が出資し2003年に完成した。
*6. KAGRA:岐阜県神岡鉱山の地下に設置された、基線長3kmのレーザー干渉計型重力波検出装置の愛称。世界でまだ誰も捉えたことがない重力波を、年に数回程度観測することを目指している。名前のKAは「神岡」のKA、GRAは重力(Grabity)や重力波(Gravitational wave)をイメージしている。